And then I wrote…
On January 12, 2021, the NASA Mars rover Curiosity marked 3000 Martian days on the surface of Mars.
In 2012 I was invited to write an article about the exploration of Mars; “Curiosity e l’esplorazione di Marte” appeared in the Jesuit journal La Civiltà Cattolica on November 17,2012. Here is the original English version that I submitted to them. As it is more than 5000 words long, I will publish it over two weeks.
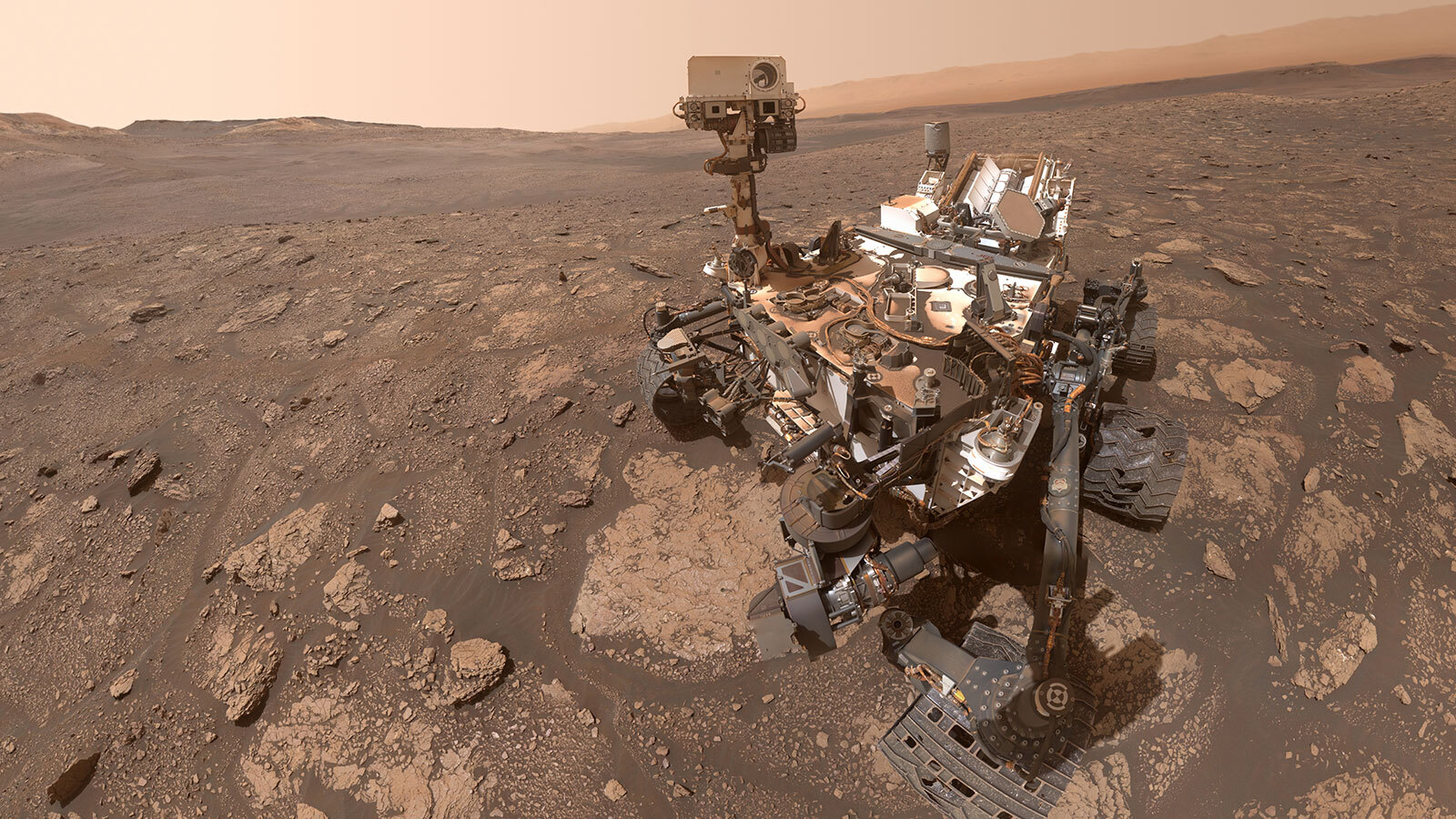
One cannot make an observation of anything in nature without, in some way, altering the very object we are trying to observe. In quantum physics, we know that if we shine even the tiniest bit of light, a massless photon, onto a particle to determine its position or momentum, the impact of that photon will nudge the particle into a different position with a changed momentum. What we thought we were observing will have become changed by the very fact of us looking at it.
The landing of a spacecraft onto a planet also makes a tiny nudge on the planet’s path through space. Of course, for all intents and purposes that change is utterly negligible. But there are other ways that it can alter the place it is trying to observe.
The Curiosity Rover, which arrived on the surface of Mars on August 6, 2012 (Rome time), is a robotic chemistry lab on wheels about the size of a Fiat 500. It is capable of traveling across the Martian surface to reach and sample rocks from many different geologic strata, looking for organic chemicals that may give us clues as to the likelihood of life now, or in the past, on that planet. The design of the mission centered on probing Mars’ surface rocks for organic chemicals; thus it has taken great pains to reduce to a minimum how much it disturbs and changes the very chemicals it is looking for, and not introduce its own organic contaminants into the Martian ecosystem.
For example, consider the unusual way it came to land on the surface of Mars. When the spacecraft reached the top of the Martian atmosphere, it was traveling at nearly 6 kilometer per second, or more than 21,000 km/hr; the gravity of Mars would only increase this speed as the lander fell toward its surface. The challenge has always been to slow such a spacecraft down to come to a gentle landing on the surface that would damage neither the lander nor the surface on which it was landing. Previous landers have used a combination of parachutes, airbags, and rockets. (The thin Martian air means that, unlike landing on Earth, parachutes alone cannot slow down a Mars lander.) But the exhaust from rockets can contaminate the surface around the landing site.
Some landers have been encased in large inflated airbags, similar to those in your car, made of Kevlar (the stuff of body armor) that “bounced” on the surface, but Curiosity was much too large to use such a landing system. Instead, it used a parachute and rockets mounted on a separate stage; beneath it dangled the lander on a 7.5 meter tether. Once it sensed that the lander had touched down, the rocket stage detached itself and flew off to crash a few hundred meters away from the rover. By keeping the rockets well above the surface of the planet, contamination from the rockets was kept to a minimum.
Curiosity is but…
[In order to read the rest of this post, you have to be a paid-up member of Sacred Space, and logged in as such!]
…the latest and largest in a long series of spacecraft sent to explore our neighbor planet. After three primitive flyby missions in the 1960s (Mariners 4, 6, and 7) and an orbiting spacecraft (Mariner 9) in 1971 imaged the surface of Mars from space, the first successful landers, Vikings 1 and 2, were set down on the surface of Mars in 1976. They were very ambitious – and very expensive – science labs designed to search directly for the presence of life in the surface soil. (In many ways the Curiosity probe is an updated version of their original design… learning lessons from what worked, and didn’t work, forty years ago.)
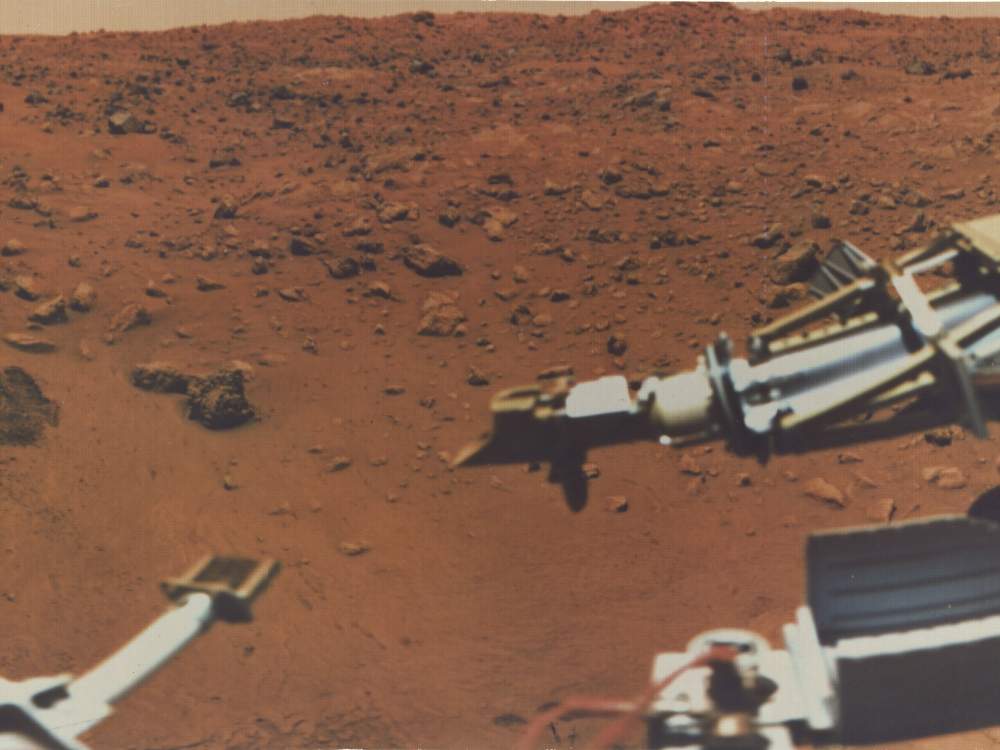
The Viking probes did not have the ability to move from their landing spots, but each did have a small robot arm that could scoop nearby soil and pour it into a series of science labs within the lander. This soil was then directed into four different experiments that could have detected evidence that would indicate the presence of life within the Martian soil.
Viking’s Gas Chromatograph/Mass Spectrometer was not itself labeled as a “biological” experiment but its results had clear implications for the detection of organic chemicals. Soil was heated to a range of temperatures that would allow different organic chemicals to boil off, and then this instrument measured the composition of the gas. It found not only that Mars soil had no organic compounds within it, but that indeed the amount of carbon in the soil was actually less than that on our airless, lifeless Moon!
After these negative results were reported, theorists realized that the Sun’s ultraviolet light (which reaches the surface of Mars much more easily than it does Earth, due to the thinness of Mars’ atmosphere) could have reacted with that thin Martian air to produce highly reactive chemicals which, when heated, would have destroyed any organic material. In fact, thirty years later, the Phoenix lander on Mars detected just such chemicals, perchlorate ions, in the Martian soil. Thus the failure to find organic chemicals in material exposed to the air was neither surprising nor necessarily indicative of what might be buried deeper in the Martial ecosystem.
The results from the three directly “biological” experiments were likewise inconclusive.
The Gas Exchange experiment added nutrients and water to the soil, and then measured the atmosphere above the soil to look for any gases produced by bacteria within the soil feeding on those nutrients. It found nothing.
The Labeled Release experiment likewise fed the soil a liquid nutrient mix where the carbon atoms were spiked with radioactive (and thus easy to detect) carbon-14. Unlike the first biology experiment, this one showed a very strong positive response: but it reported a spike of carbon-14 released as soon as the nutrients hit, not the gradual release that one might expect from soils. One theory suggested that, again, solar UV had made the soil especially reactive in the presence of water, but similar results were found whether the soil came from the surface or under a Martian rock. More oddly, when the result was repeated a week after the first trial, no gas was released. For nearly forty years, this result has remained controversial. Some modelers insist that it is actually quite consistent with the presence of life, but most scientists are skeptical.
The final experiment on Viking separated the soil into two halves, sterilized one, and then exposed both halves of soil to carbon-14 gas. After waiting for any microbes (in the unsterilized half) to incorporate the gas, the soil was then tested to see how much carbon-14 it had taken on. If there was a strong difference between the sterilized and unsterilized samples, one could conclude that some sort of microbes were responsible for that difference. In fact a small difference was recorded; but it was so small that one could not be sure it was not just due to random fluctuations within the experiment from run to run.
Besides these experiments, the Viking landers had cameras that took images of the nearby terrain, and orbiting cameras that mapped the entire surface of Mars. Again, the results of these were ambiguous when it came to the search for life. The landers showed a barren red desert, strewn with rocks; no life was visible, nor could one imagine even the hardiest plant surviving there. The orbiters, however, revealed that much of Mars was criss-crossed with terrain that looked like it had once been covered with flowing water, at least in some distant past time.
From the point of view of understanding Mars as a planet, the Viking program was a great success. The images from space provided the detailed maps of terrain and geology on which all future understanding of the planet, and all future missions, have been based. The surprising if ambiguous chemical results inspired a far more creative way of understanding the complex chemical relationships between surface material, atmosphere, and solar radiation. And the images from the surface transformed, in our imaginations, the concept of Mars from a point of light in a telescope, or a ball in space, to a place where we could imagine ourselves (or at least our robots) roaming about. Mars became a place where we could imagine adventures taking place.
But the Viking mission had been sold to the public, and the American government that had paid for it, as a search for life. And in that regard, it was a failure.
Indeed, it was a remarkably expensive failure from that point of view. The Viking mission cost $935 million in 1974; considering inflation, that corresponds to nearly $4.5 billion in 2012 dollars, making it the single most expensive robotic mission ever flown to a planet. It was designed to give a solid, yes-or-no answer to the presence of life on the surface of that planet. But even though all its experiments worked as designed, it failed to give any such definitive answer.
That failure, in turn, resonated through the field of planetary science. It would be twenty one years before another robot was sent to the Martian surface.
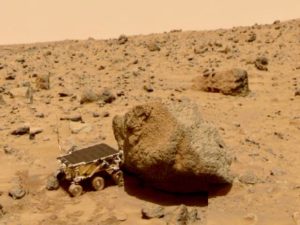
In 1997, a very different sort of mission arrived on Mars. Only half the mass of the Viking probes, Mars Pathfinder included both a fixed lander and a small roving vehicle with a probe to do a basic chemical analysis of the rocks it encountered. Because of its small size, it was able to land (after using a parachute and rockets to slow its decent) by inflating several dozen airbags about itself and then bouncing along the surface until it came to rest. (The first bounce lofted it more than 15 meters above the Martian surface; it bounced another 15 times before rolling to a stop, at which point the airbags were deflated and the spacecraft unfolded and righted itself.)
Along with this audacious arrival, it carried an equally rakish little rover, a 10 kg solar-panel-on-wheels that, frankly, looked cute. Watching it trundle up to rock after rock and make its basic chemical tests, the public was inevitably reminded of the playful little robot, R2D2, from the popular Star Wars movies. In a competition open to the public, it was given the name “Sojourner.” A star was born.
In addition to the robot, the lander itself carried a camera system that benefited from 20 years’ advances in imaging and computer technology over what Viking could carry. The resulting panoramas from that imager, including three-D views, provided a far more realistic view of the surface than anything Viking had achieved.
The lander imaging team had its own impish sense of humor, beginning with its name: “Imager for Mars Pathfinder”, or IMP. It continued the mission’s engaging take on the science by informally giving the various rocks visited by the rover names of children’s cartoon characters.
This was, in fact, not a clever public relations ploy but something of an accident. In order to keep track of which rock was which while planning the path of the rover, the first two rocks near the lander were identified by names based on what they first looked like to the team. One rock covered with small lumps that looked like barnacles was called “Barnacle Bill”, a character in an old drinking song that had been used in a Betty Boop cartoon in the 1930s. The other, a triangular shaped rock, was thought to resemble the head of the 1960s cartoon character Yogi Bear.
That evening, the chief imaging scientist phoned his family in Arizona from the Jet Propulsion Laboratory in California, where the team was working. His five-year-old son begged him to name another rock for his own favorite cartoon character, Scooby-Doo. The following day, that name was added to the list; once the pattern had been set, all further rocks bore cartoon names. The Cartoon Network, a cable television network in the US that owned the copyrights to these character names, was so delighted that they sent a box of tee-shirts with the various characters printed on them as a gift to the imaging team. (The boy who asked for Scooby-Doo is now a scientist himself.)
Adding to its charm in the eyes of the US Congress was that the Pathfinder mission had spent only a tiny fraction, about 5%, of what the Viking program had cost.
Meanwhile, another major development had occurred in our understanding of Mars between the time of the Viking landings in 1976 and the Pathfinder success in 1997. In the early 1980s, a convincing number of different lines of evidence had succeeded in convincing most scientists that a particularly rare clan of basaltic meteorites (there were only a dozen known at that time) were in fact samples of Mars itself. The prime examples of meteorites in this group were Shergotty, which fell in India in 1865; Nakhla, which fell in Egypt in 1911; and Chassigny, which fell in France in 1815. Thus these meteorites are often called the “SNC” group.
The first suggestions that these meteorites were Martian had been made on the basis of the ages and chemical compositions of these rocks.

Most meteorites come from tiny, primitive asteroids, and were formed where oxygen was scarce; they contain iron metal and traces of hydrated carbon. But the iron in the SNC meteorites is highly oxidized; instead of metal, it is rust. And some SNC meteorites contain carbonate minerals, compounds of carbon and oxygen. This would be consistent with them coming from a body that had an atmosphere, in particular a carbon-dioxide rich atmosphere like Mars.
The next line of evidence had to do with their formation ages. Most meteorites have not been altered chemically since they were formed at the beginning of the solar system, 4.6 billion years ago; and even the youngest Moon rocks were known to be at least 3 billion years old. However, the accumulation of the decay products from radioactive elements in meteorites of the SNC group indicated that many of them crystallized from molten lavas only about a hundred million years ago, making them thirty times younger than the Moon rocks. Simple physics tells us that the larger a planet, the longer it can stay warm enough to melt rocks in its interior. If even the Moon (which is much larger than any asteroid) froze up three billion years ago, then the SNC basalts must have come from a body larger than even the Moon. Mars is the next largest candidate available in our solar system.
But the key link in this chain of evidence connecting these samples to Mars came when a meteorite chemically similar to Shergotty, recovered in Antarctica in 1979, was found to have a glassy bit of frozen lava that contained tiny bubbles of gas. When the composition of the air in these bubbles was measured (the sample was melted inside the vacuum chamber of a mass spectrometer, which identified, atom by atom, the mass of the each atom released) it was found to match precisely the very unusual composition of the atmosphere of Mars… a composition that had been measured by the mass spectrometers carried aboard the Viking landers.
How were the meteorites actually were able to get launched off Mars? That’s a hotly debated point which is still not resolved to everyone’s satisfaction today. The best models suggest that it takes an unusual coincidence of a rare impact of a small asteroid into Mars at a place where the Martian geology provides just the right conditions for material to be launched off the surface, fast enough to escape Mars’ gravity, without actually destroying the launched rocks themselves. Thus, while these may be samples of Mars, they may well not be particularly typical of Mars rocks as a whole.
Then, in 1996, another meteorite from Antarctica that appeared to be isotopically related to the SNC group was found to contain traces of chemicals and minerals that some scientists argued were evidence of ancient fossilized microbes. Other scientists disputed this interpretation of the data; but the very suggestion was intriguing enough that the whole issue of life on Mars was reopened.