And then I wrote… this is the second half of the article I started last week, originally published in Italian in Civiltà Cattolica; this is the original English text. While much has developed since this article was written — see the links inserted here — I think the questions I raised then are still valid, and it’s also fun to see what we were thinking about Mars, almost ten years ago…
The confluence of this “Mars life” rock [in 1996] and the popular Pathfinder mission [in 1997] gave both a scientific and political motivation to begin a systematic search for life on Mars. Unlike the previous Viking missions, however, this new program would proceed in a series of smaller steps.
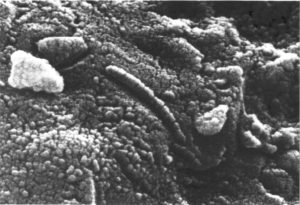
Viking’s major mistake had been that its design assumed too much about the sort of life it expected to find and the environment where it expected to find it. It was, in essence, a robot designed to find terrestrial life on Earth; it was not at all prepared to find life in the very different environment of Mars. It had landed at sites chosen to be easy to reach with a lander, not necessarily those where traces of life were likely to be found. It had assumed that life traces would be evident on the surface of Mars, rather than more deeply hidden and protected from the harsh solar ultraviolet light and cosmic rays. And it had assumed that Mars life would behave chemically in essentially the same way as terrestrial life did.
Instead, armed with the failure of the Viking search, the mission planners realized that one needed to know more about Mars before a proper search for life within Mars could be launched.
(Indeed, one needed to know more about life, as well. Thus, parallel to the Mars missions, an ambitious program in astrobiology was also begun. One of the primary threads of this research was to look for, and understand, “extremophiles”: microbes on Earth that lived in extreme environments such as hot springs, acid lakes, and regions of high radiation. As a result, we are now aware of lifeforms that thrive deep within the Earth itself, under conditions where life might also be able to exist on Mars.)
The program design had three stages. First, along with developing a more general understanding of Mars’ geological history, we’d look for the places on Mars where water was easy to find. Next, once the appropriate technology was developed to get a spacecraft to those regions, we’d land there and look for traces of chemicals that might be indicative of biological activity. And finally, once we knew what to look for, we’d figure out a way of taking an appropriate sample from such a region back to Earth, where we could study it at leisure with all the tools available to us here.
[In order to read the rest of this post, you have to be a paid-up member of Sacred Space, and logged in as such!]
The first stage, understanding Mars and looking for the water, led to a series of space missions launched every two years (when the orbits of Earth and Mars are best aligned for sending probes). The Mars Global Surveyor had arrived at the planet the same year as Mars Pathfinder, giving an updated geological survey of the planet’s surface topography; the NASA Mars Odyssey orbiter, launched in 2001, mapped the surface mineral composition. In 2003 the European orbiter, Mars Express, and NASA’s Spirit and Opportunity rovers were launched to explore the surface in more detail. Using the results of the earlier orbiters and rovers, the Mars Reconnaissance Orbiter was sent in 2005 with detectors keyed to search for particular minerals, like carbonates, symptomatic of the presence of water. It included a detector that could map in detail the locations on the surface rich in hydrogen (the “H” of “H2O”), indicating the places where water ice might be found not too far beneath the surface sands. Then the 2007 NASA Phoenix mission landed in one such region in the Martian far north, and uncovered water ice just a few centimeters below the surface, confirming the results of the orbiters. With the success of these missions, Curiosity now represents the next stage in Martian exploration.

This litany of successful missions should not imply that getting to Mars is easy. According to a NASA web site that keeps tab on these things, there is an even longer list of Mars missions that have failed. These include two attempts by the Soviets to fly by Mars in 1960, two more failed flyby missions and a failed lander by them in 1962, unsuccessful US and Soviet flyby attempts in 1964, two Soviet attempts in 1969 that failed at launch, an American failed launch in 1971 along with three Soviet failures that year, and four failed Soviet missions in 1973. Between Viking and Pathfinder, three other attempts to reach Mars by the Soviets (in 1988) and NASA (in 1992) ended in failure. After Pathfinder, in 1999 there was a failed US mission and a mostly-unsuccessful Japanese mission; the ill-fated Beagle lander from the Europeans in 2003; and a joint Russian-Chinese mission launched in the fall of 2011 that failed to leave Earth orbit. In some cases, the missions listed above blew up on launch or malfunctioned en route to Mars. Others crashed on the Martian surface or missed Mars entirely. A few of them arrived safely but sent back only a tiny fraction of the hoped-for data before instrument malfunctions ended their useful life.
In this context, one can understand the complex design of the Curiosity rover. Unlike the earlier missions, it knew exactly where it wanted to look, and so it had a precise landing spot, targeted in Gale Crater. The spacecraft’s outer shell was designed to allow it to steer itself through the atmosphere as it came down, to arrive to within 2000 meters of the target center. The complex “skyhook” rocket system not only kept the rocket exhaust away from the landing site, it also allowed the spacecraft to land at a higher altitude (and thus in thinner air) than previous landers. And the rover was designed to climb up hills and over rocks to reach specific outcrops that had already been mapped from orbit and were marked for special study. Furthermore, Curiosity is powered by a small generator using the heat of a radioactive element to provide the electricity that drives it wheels and runs its chemical labs; this insures that its power is not affected by dust storms, seasons, or the time of day, unlike the solar cells that powered earlier missions.
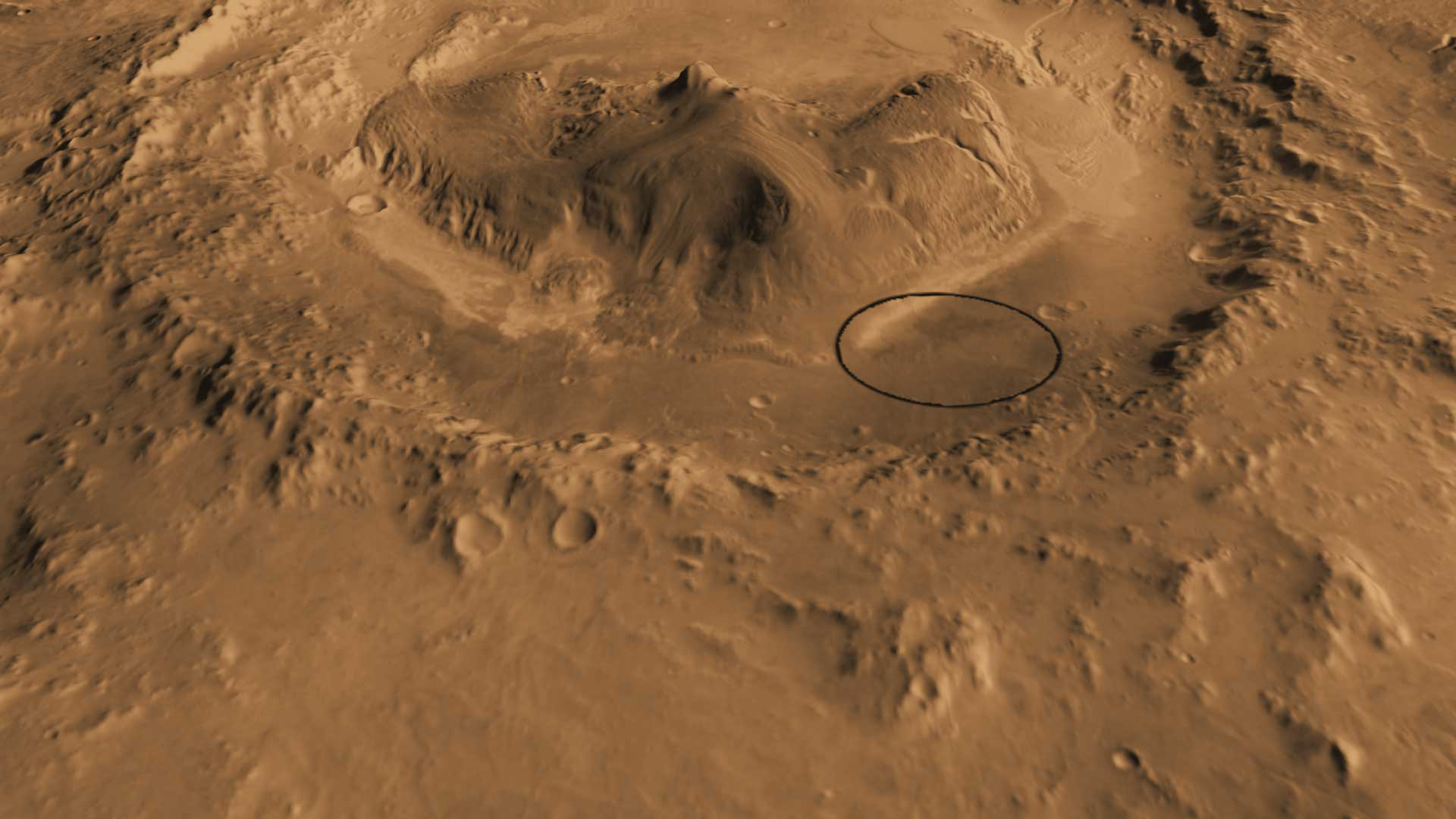
All of its navigation is done in real time by on-board computers. Pilots back on Earth can’t guide it, because Mars is so far away. Even a signal traveling at the speed of light can take ten to twenty minutes to go from a camera on Mars to a monitor on Earth, and then another ten to twenty minutes will pass before our response on Earth can get back to the rover on Mars. This ability of the rover to think for itself, both when it flew towards its target during its landing or now as it dodges bounders on the surface, requires computers and software that would have been impossible to imagine in the days of the Vikings.
Curiosity’s scientific mission is no longer to look for evidence of water alone, but to sample organic chemicals that are most likely associated with such water. Its target areas are a series of cliffs excavated when Gale Crater, its landing site, was formed. By looking at outcrops like these, we hope to be able to sample material that spent most of its geological history buried well below the surface and thus more likely to have provided a sheltered environment for life to have developed. We don’t expect to see life itself – if it had been there, it would almost certainly be destroyed now that it is exposed to the surface – but rather the trace chemicals left behind from where it once might have flourished.
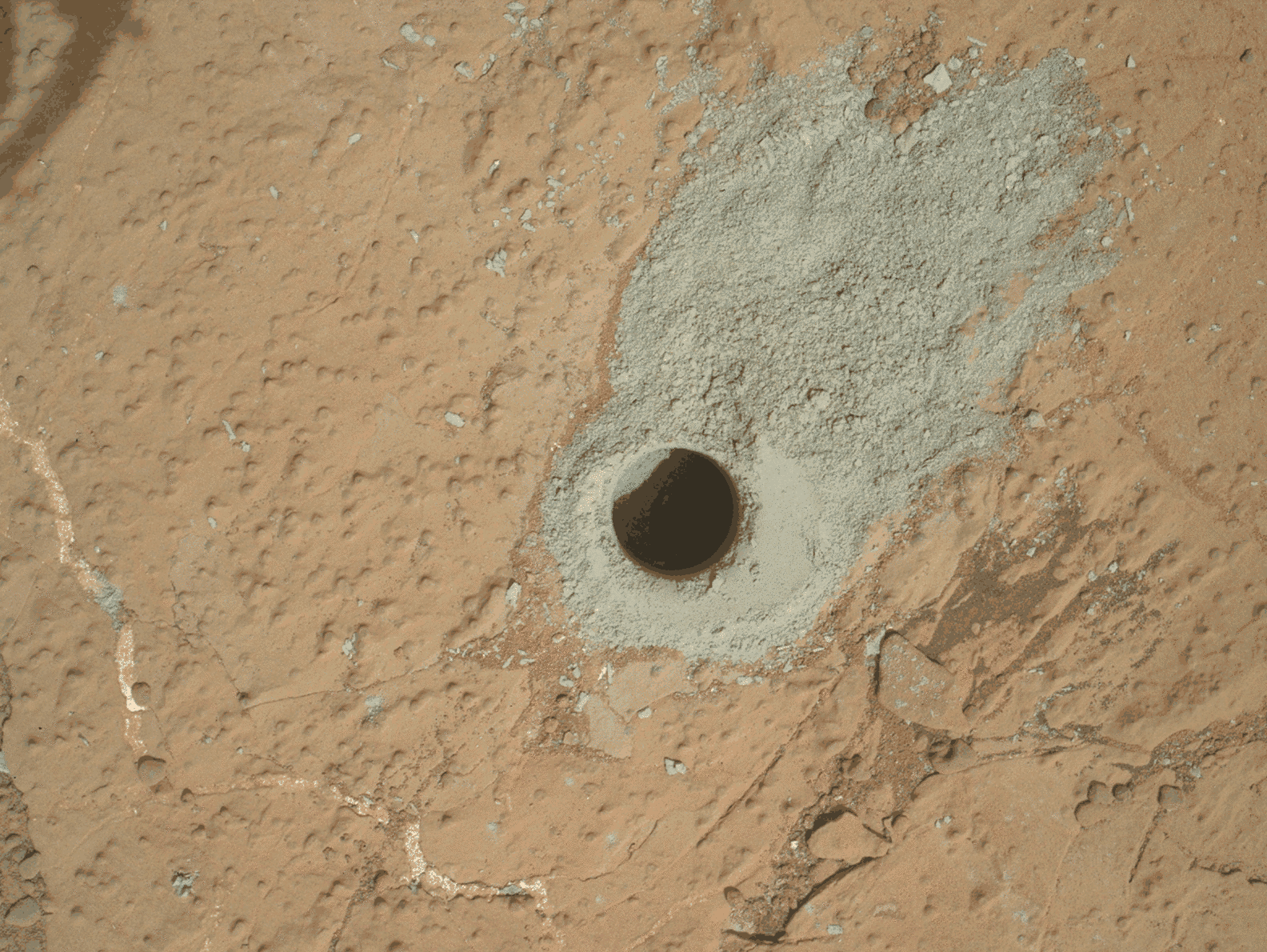
To this end, the probe includes a drill that can penetrate a five centimeters into Martian rock. Calculations show that solar radiation and cosmic rays should effectively sterilize material in the first two centimeters of the Martian soil, but there is hope that deeper down one might still find organic material intact. Unfortunately, after the drill bits had been sterilized and sealed in their box on the rover, just before its launch a late decision among the mission managers re-opened the box and inserted one of the drill bits into the drill. (They were worried that the box itself might became damaged and couldn’t be opened after Curiosity landed; they didn’t want to have a drill with no drill bit.) This means that, since the box was re-opened on Earth, the bits are no longer certified as sterile. Care must be taken, therefore, to insure that they do not actually touch ice or water on Mars where terrestrial microbes could potentially infect the planet… and ruin any chance of us detecting the unambiguous presence of purely Martian life. (Wet soil would clog up the drill bits in any event.)
In addition to the soils, Curiosity’s mass spectrometer will also sniff for methane and other organic compounds in the Martian atmosphere. A few years ago, a controversial detection from Earth-based telescopes suggested that clouds of methane might be found intermittently in the atmosphere. Since methane is produced by biological processes on Earth, yet would not survive for long exposed to sunlight at Mars, its presence was hailed as evidence of life existing there today, buried below the surface of Mars. However, other observers have strongly questioned this evidence. Seeing any spectra of methane on Mars requires a difficult and tricky bit of data reduction, since light from the air of Mars must also pass through Earth’s atmosphere, with all its trace gases, before we can capture it here with our telescopes. The Curiosity probe should be able to determine if this gas is really there, and if so how abundant it is… and if it looks, in detail, like the kind of gas emitted by microbes.
The Curiosity mission should be completed by then end of 2014; its power source is good for a Martian year, which is roughly two Earth years. [In fact, Curiosity’s two-year mission was extended indefinitely in August of 2017; and its power source has a minimum lifespan of 14 Earth years. As of 2021 it is still going strong, and updates on what it is up to can be found here.] What comes next?
The next NASA mission to Mars, MAVEN (Mars Atmosphere and Volatile EvolutioN), is scheduled to launch in November of 2013. [It did, on November 18 of that year.] It is an orbiter, designed to skip the top of the Martian atmosphere and measure how quickly gases leak off into space. [It was a success, and has been in orbit around Mars for six years as of this writing] Recall that images of the surface of Mars suggest that the planet was once much warmer and wetter, but the current atmosphere is so thin that any water were exposed on the surface today would immediately evaporate and not remain in a liquid form. The ancient atmosphere must have been thicker. This mission will test whether the gradual loss of atmospheric atoms against Mars’ lower gravity is enough to account for all the air it lost, or if one must evoke more exotic events (like the giant impact of an asteroid into Mars) to remove its ancient atmosphere.
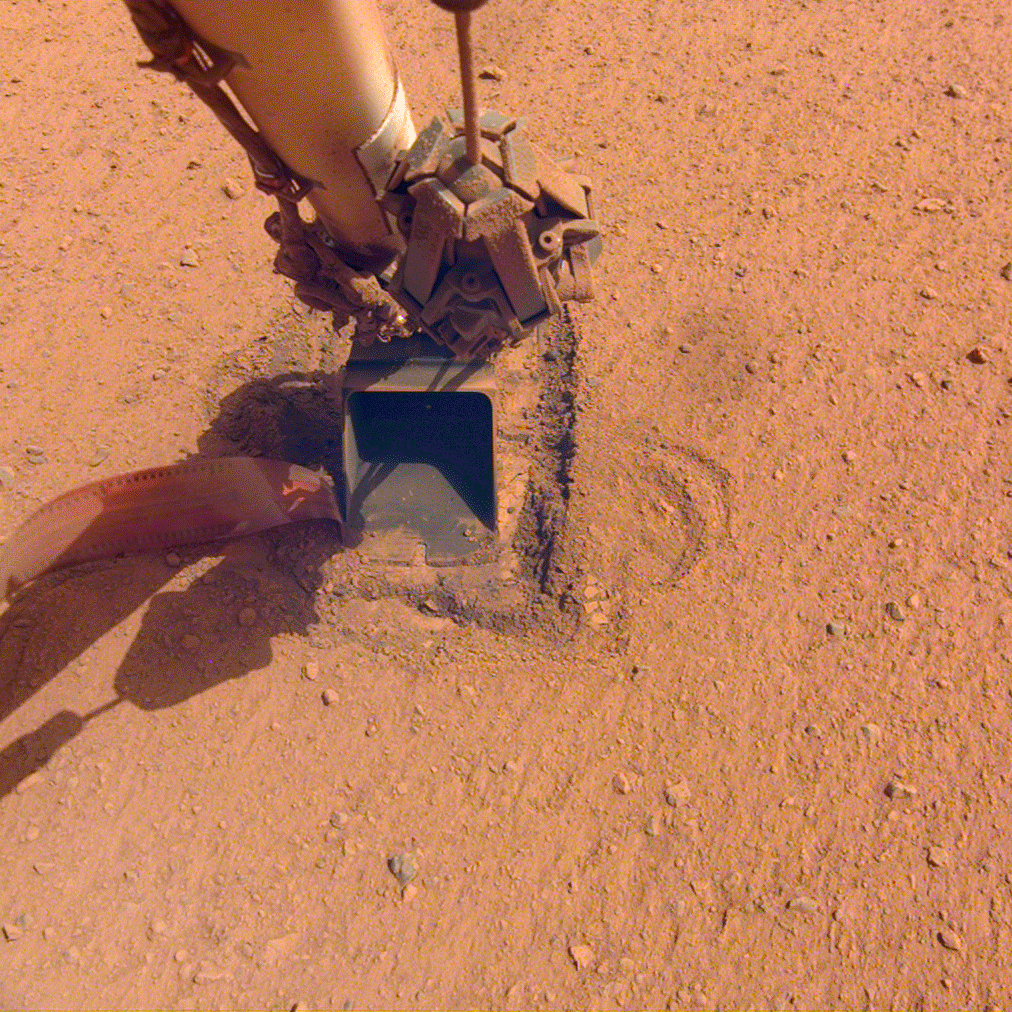
Most recently, NASA has just approved another Mars mission, “InSight,” to arrive on the surface in 2018. [It did.] It re-uses the same spacecraft system as the 2007 Phoenix lander, but this time carrying a seismometer to detect marsquakes and a probe to measure the amount of heat coming out of the planet. In this way, we hope to get more detailed information about what the interior of Mars is like. Besides doing interesting science in its own right, InSight may also tell us something about places below Mars’ surface where life may be hiding. [Another success, it has been sending us back data from Mars for the last two years. Alas, its mission to drill deep into Mars has been abandoned; the surface was harder to crack than had been expected.
Beyond those modest missions, however, the future of Mars exploration is quite uncertain. The original dream was to use the results of Curiosity to learn what sorts of samples on the Martian surface looked to be especially intriguing and then send a series of rovers to gather such samples into a stockpile at some easy-to-reach location on the surface. The next step would then be to land a spacecraft next to those rocks, load them up, and launch them back to Earth. But the technical challenge of building such a mission, not to mention its cost, has put that program in doubt.
[The next rover survived the budget cuts; the Mars Perseverance rover was launched on July 30, 2020, and lands on Mars February 18, 2021. For more details on its planned activities, click here.]
Part of the difficulty has come from Curiosity itself. The mission was originally supposed to arrive in 2010 and it was first budgeted at about $700 million, but soon cost-overruns nearly doubled its budget to $1.6 billion. By the time it finally launched, it was two years late and cost $2.6 billion, nearly two billion dollars over its original estimate. This cost overrun ultimately led NASA to cancel its participation in the European ExoMars mission, a billion-Euro mission with a rover and orbiter designed as a follow-on to Curiosity. With Russian participation, the European Space Agency’s ExoMars lander arrived on Mars in October of 2016; but the loss of NASA support has certainly hurt the mission.
Indeed, Curiosity may signal the beginning of the end of our current cycle of exploration on Mars. It may be another two decades, or more, before resources once again become available to try and understand the only other planet besides Earth where one could expect to send human beings. (Mercury and Venus are too hot, and the moons of the gas giant planets too cold and distant, for any human visits any time soon.) Curiosity, the spacecraft, has taken great care not to alter or poison the physical environment it wished to study on Mars. But Curiosity, the mission, has seen its cost overruns poison our ability to study the solar system in the near future.
And in fact, Curiosity’s cost, bad as it was, is nothing compared to that of NASA’s replacement for the aging Hubble Space Telescope. The James Webb Space Telescope was originally slated to cost $1.6 billion and launch in 2011; the best estimate now puts its price tag is an astounding $8.7 billion and it won’t be launched until 2018 at the earliest. [Currently it is scheduled to launch in October of 2021, with a budget now estimated to run over $10 billion.] This overrun has robbed funds from every other program at NASA, including ground-based astronomy and lab work as well as other space missions. In an ominously familiar way, it has become a program “too big to fail”… too big to cancel. So instead it has led to the canceling of a lot of other proposed space missions.
On the other hand, the discoveries and images Curiosity returns to us may yet surprise us. Some new revelation about life, some adventure on its surface, some awe-inspiring image of Martian vistas, may yet inspire us to look again at how we wish to spend the tiny fraction of our resources that such space missions entail. (Simply type “$2.6 billion” into Google and see how many mundane projects, from business deals to government bailouts, are routinely covered at that cost. For example, that’s how much Coca Cola spent in advertising in 2006.)
And even as it works to preserve the nature of the planet it is studying, Curiosity will inevitably change the “Mars” that exists in the human imagination. The more we find traces of life – or the absence of life where one might expect it could survive – the more we will alter the way we living creatures understand life itself, and what it means for us to be alive. Perhaps beyond changing what we imagine, it will also lead to changes in the human imagination itself. Curiosity may never be the same.
[In fact, four missions to Mars were launched in 2020, with more planned for 2022. Keep tuned!]